Physics’ Claudia Ratti Weighs in On Future of Quark-Gluon Plasma Research
In the standard model of particle physics, matter is held together by four fundamental forces. Depending on the scale, these forces are what hold together entire solar systems, or the particles that make up protons and neutrons.
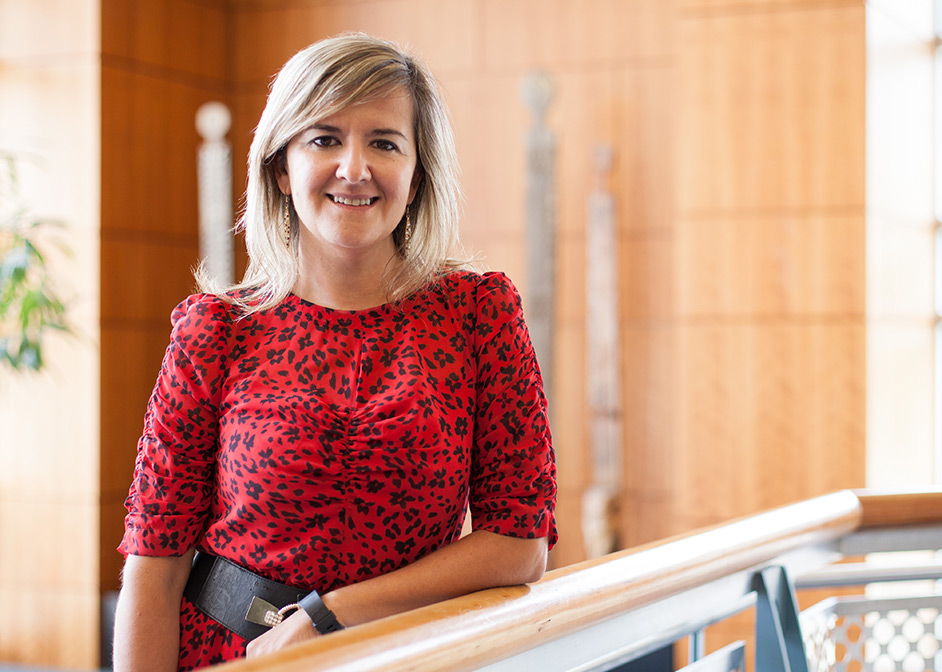
The Strong Nuclear Force: Binds Together Quarks and Gluons
Claudia Ratti, associate professor of physics, studies the strong nuclear force, which binds particles known as quarks and gluons into the protons and neutrons that make up atomic nuclei. Understanding how this force works is a challenge, requiring big efforts, both in experimentation, as well as theory.
Recently, in a review for the journal Reports on Progress in Physics, Ratti weighed in on the different roles theory and experiment play in pushing the boundaries of our understanding about the strong nuclear force. Ratti started her career as a phenomenologist, someone who looks at data to explain natural phenomena. She later shifted into fundamental theory, making her uniquely suited to bridge the gap between the two.
“Theory alone or experiments alone will not be enough. In order to understand such a complex system, we need the synergy between fundamental theory and experimentation,” Ratti said.
Both Theory and Experimentation Needed
Within the past few decades, with the increase of computing power and the development of large-scale experiments, the possibility of understanding this force is becoming more of a reality.
“The theoretical framework can only be solved numerically, with the codes needed to solve it running on some of the most powerful supercomputers in the world,” Ratti said. “In the past, the algorithms we were using, and the computational power we had available, were not enough to get realistic results.”
With the advent of supercomputing facilities at the exa-scale and the development of parallel processing software, the tools to make quantitative predictions for the creation of matter in the Universe are now available.
On the experimentation side, the construction of heavy ion colliders, such as the Large Hadron Collider at CERN, as well as the Relativistic Heavy Ion Collider at Brookhaven National Lab, are ushering in a new era of experimental evidence.
These colliders smash together heavy ions, such as gold or lead, creating quark-gluon plasma, in which protons and neutrons melt into their fundamental particles, swimming in a ‘particle soup.’
Advances in Particle Physics
As much as the field has advanced within the past few decades, there are still many unanswered questions. Current methods in computation require theoreticians to work with approximations, simulating quark-gluon plasma in a state of equilibrium.
Experimental evidence from the colliders measures the formation of quark-gluon plasma, which is achieved for only the tiniest fraction of time. These measurements record a state of change, from protons and neutrons to quarks and gluons, and then back to protons and neutrons.
“These experiments are a very dynamical process,” Ratti said. “To make the connection between experiments which are highly dynamical and simulations which model static conditions, you need to find the right observables that would make sense to compare.”
- Rachel Fairbank, College of Natural Sciences and Mathematics